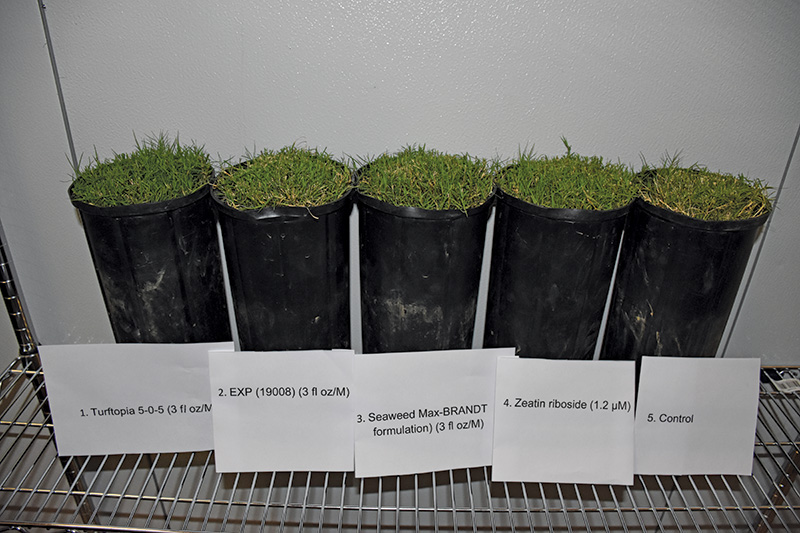
Figure 1. Effect of treatments on turfgrass color and vigor after chilling stress (Aug. 25, 2021). Photo by Xunzhong Zhang
Bermudagrasses and other warm-season grasses planted in more northern and colder climates, particularly the transition zone, are susceptible to reduced performance and winter injury. The optimum temperature range for bermudagrass shoot growth is 81 to
95 F (27 to 35 C), with maximum tillering occurring at temperatures slightly below the optimum shoot growth temperature (2). As expected, soil temperature has a greater influence than air temperature on bermudagrass root growth, with the ideal range
from 75 to 84 F (24 to 29 C) (1). Bermudagrasses, particularly fine turfgrass varieties maintained on golf courses, are rather cold-sensitive and are susceptible to chilling stress below 54 F (12 C) (2). Chilling stress of warm-season grasses has
been characterized by a necrotic lesion, loss of chlorophyll, reduction in photosynthetic rate and slowed growth (2). Chilling stress often leads to secondary and/or tertiary stress, such as oxidative stress or increased pest pressure. Selecting a
more cold-tolerant hybrid is an option, but renovation may not be feasible.
Tolerance refers to a plant’s resistance to temperature stress resulting from the prevention or reduction in injury from that stress (5). Bermudagrasses respond to chilling stress by altering cell membrane properties, accumulating cellular compatible
solutes (osmolytes) and increasing antioxidant production (9). Cultural practices designed to improve plant vigor — such as mowing height adjustment, judicious nitrogen use and correct fertilization strategies, and improving drainage —
will offer plants better tolerance to stress. But what about routine applications of elicitors designed to further enhance this response? Exogenous application of these “stress reducers” may offer turfgrass managers a competitive edge
when maintaining bermudagrasses in the transition zone.
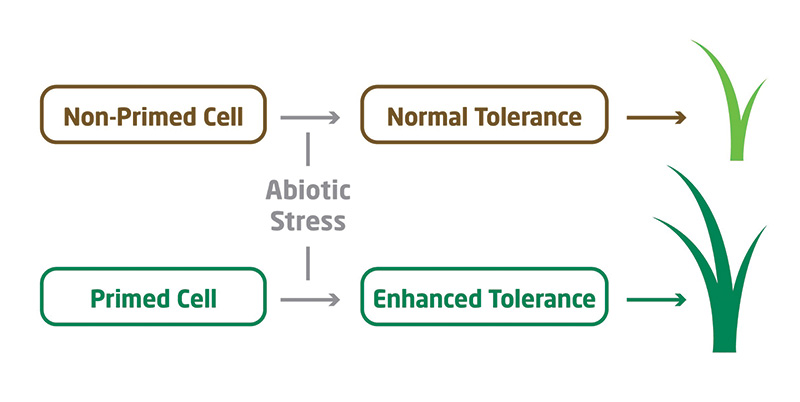
Figure 2. Primed plant cells, either by acclimation or treatment with elicitors, will offer plants enhanced tolerance to abiotic stress.
Elicitors, previously known as biostimulants or biochemicals, upregulate a plant’s already existing natural defense mechanisms. Cells primed for stress allow plants the luxury of better stress tolerance (Figure 2).
Elicitors have been studied for their use as part of effective turfgrass programs for many years, however most of this work was conducted on cool-season grasses. Examples of elicitors most studied include seaplant extract (Ascophyllum nodosum), humic
acids, amino acids, vitamins, antioxidants and phytohormones. Cytokinin applications, natural (Ascophyllum nodosum) and synthetic, have been shown to increase creeping bentgrass root viability when exposed to heat stress (6, 7, 8). The science has
shown that the tolerance response can be largely attributed to increased antioxidant production (11, 12). In addition, amino acid and hormone applications have been shown to affect primary metabolic responses, such as increased photosynthetic activity
of perennial ryegrass after heat stress (3, 4).
The priming process that increases plant stress tolerance may come from acclimation through natural selection, breeding improvements, or from routine and sequential applications of elicitors, or any combination of those processes. Previous research has
shown that the effectiveness of exogenous elicitor applications largely depends on making multiple treatments prior to the onset of stress (3, 10). Limited research could be found that tested the effects of elicitors on warm-season grasses and their
subsequent tolerance of chilling temperature stress.
A greenhouse study was designed to determine: 1) how cold temperatures impacted bermudagrass growth and development, including antioxidant production, 2) the impact of elicitors on bermudagrass quality, color, photosynthetic capacity and antioxidant concentration
after prolonged exposure to chilling stress, and 3) if treatment with the commercially available formulations differed from the synthetic hormone treatment (industry standard).
Materials and methods
The two-year study was set up as a randomized complete block design with four replications on hybrid bermudagrass (Cynodon dactylon x) var. Latitude 36. The bermudagrass was established from sprig in plastic pots (5-inch diameter, 12-inch depth; 13 centimeters
by 30 centimeters) consisting of a 90% sand and 10% peat root-zone mix. Plants were adequately fertilized to promote growth and vigor, hand-watered and cut to 1 inch (2.5 centimeters) in a growth chamber with optimum growing conditions.
Treatments were:
- Turftopia (Brandt/Grigg; 5-0-5 manganese, zinc + osmoprotectants, glutathione, salicylic acid) — 3 fluid ounces per 1,000 square feet (9.5 liters per hectare).
- Seaweed Max (Brandt; 25% Ascophyllum nodosum, 1% humic acid); Ascophyllum nodosum contains plant phytohormones auxin and cytokinin — 3 fluid ounces per 1,000 square feet.
- Zeatin riboside — 1.2 micromolar (µM).
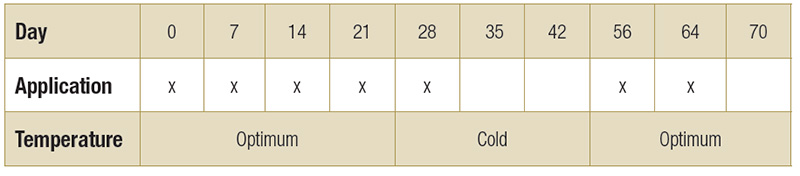
Table 1. Application schedule of treatments prior to, during and after cold stress.
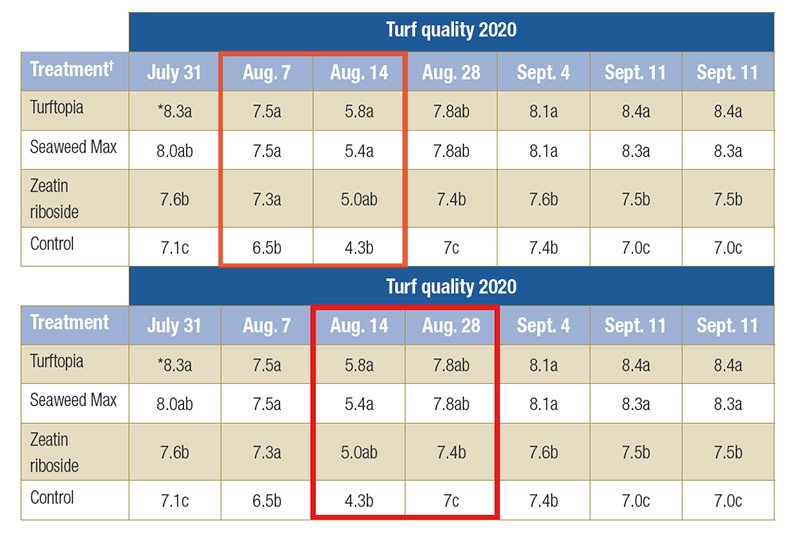
Table 2. Overall turf quality of hybrid bermudagrass before, during and after chilling stress on a scale of 1-9, where 9=best quality. The highlighted rows were data collected during chilling stress.
†Turftopia and Seaweed Max applied at a rate of 3.0 fluid ounces per 1,000 square feet; Zeatin riboside applied at 1.2µM
*Means followed by a different letter are significantly different (P=0.05)
Treatments were applied with a CO2-powered boom sprayer using three flooding nozzles at 40 psi. Cytokinin content in Seaweed Max was determined using standard radish cotyledon expansion method with zeatine riboside (ZR) as the standard. Thus, the ZR dose
was 1.2 micromolar to match the cytokinin levels in the Seaweed Max. Fertilizer inputs were calculated so that all treatments delivered identical amounts of nitrogen (N) and potassium (K), 0.01 pounds per 1,000 square feet (57 milligrams per square
meter) for each nutrient.
All pots received a Hoagland’s solution (one-half strength) routinely starting at transplanting and every seven days to ensure adequate nutrition and to not limit plant growth and development. The amount of Hoagland’s solution was designed
to supply 0.1 pounds nitrogen per 1,000 square feet (4.88 kilograms per hectare) per two weeks.
Treatments were applied sequentially every seven days for one month prior to cold stress (growth chamber). The plants were subjected to cold stress: 50 F (10 C) for 10 hours, 39 F (4 C) for 14 hours for 14 days. The treatments were applied for two additional
weeks after removal from cold treatment, and data was collected during recovery period (Table 1).
Response variables
Turfgrass quality and leaf color were rated on a visual scale of 1 to 9, with 9 indicating the best, every seven days. Clipping yield (fresh weight) was measured every 14 days. Photochemical efficiency — ratio of variable fluorescence (Fv) to maximum
fluorescence (Fm); Fv/Fm — was measured using a standard chlorophyll fluorometer (OS-50II) every seven days. Plant tissue was collected every 14 days, and antioxidant activity was measured, including superoxide dismutase (SOD), glutathione reductase
(GR), glutathione (GSH) and polyphenols. Data was collected over two years in 2020 and 2021, analyzed using an analysis of variance, and mean separations were performed using the Fisher’s protected least significance difference test at a 5%
probability level.
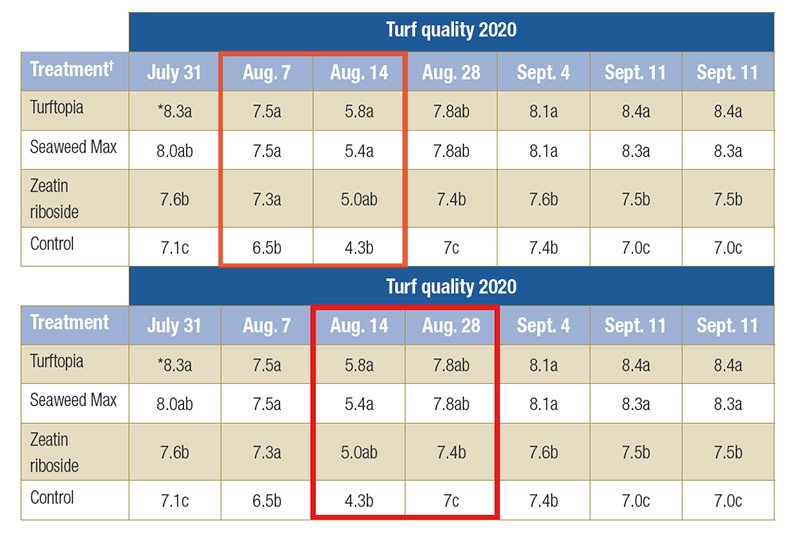
Figure 3. Effect of treatments on turfgrass color before, during and after chilling stress in 2020 on a 1-9 scale, where 9=best. The highlighted area includes the time plants were exposed to chilling stress. Statistical significance between treatments (P=0.05).
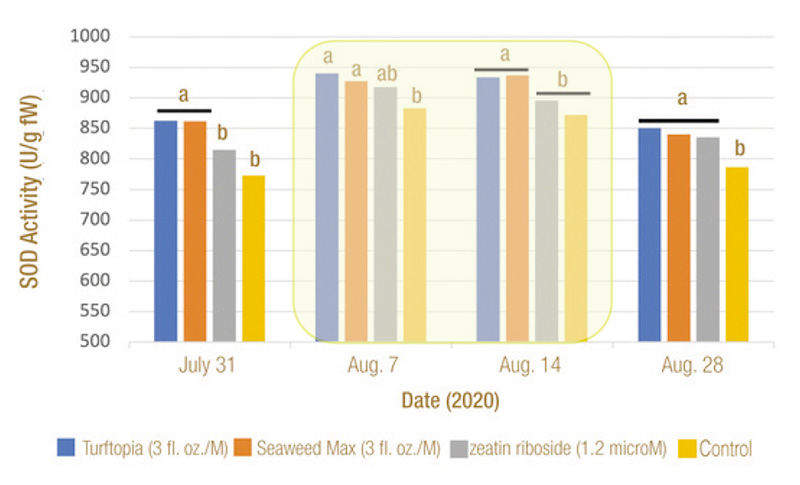
Figure 4. Effect of treatment on superoxide dismutase (SOD) concentration in bermudagrass leaf tissue in micromols per gram (fresh weight) on four rating dates in 2020. Chilling stress exposure occurred for 14 days (Aug. 1 to Aug. 14) as indicated by area shaded in yellow. Means followed by a different letter are significantly different (P=0.05).
Results
Overall turfgrass quality and shoot growth
Hybrid bermudagrass overall quality declined after exposure to two weeks of chilling temperatures. In 2020 and 2021, the two weeks of chilling temperatures reduced quality and shoot growth. This effect was most pronounced in 2020 compared to 2021. Shoot
growth was largely unaffected by treatments in both years (data not shown).
Turftopia and Seaweed Max produced the best bermudagrass quality during chilling, and differences were often significant from the zeatin riboside and control turfgrass (Table 2). In addition, bermudagrass treated with Seaweed Max tended to perform better
than bermudagrass treated with ZR, including significant differences on five rating dates, indicating that the naturally occurring form of cytokinin, Ascophyllum nodosum, produced a better chilling tolerance response than ZR in hybrid bermudagrass
(Table 2).
Overall turfgrass color and photosynthetic efficiency (PE)
Bermudagrass color response was similar to overall quality, where ratings dropped after exposure to chilling stress. However, in both years Turftopia and Seaweed Max treatments produced the best bermudagrass color just before, during and after chilling
stress. Again, this effect was most pronounced in 2020 (Figure 3).
In 2021 at the end of chilling treatment (Aug. 25), Turftopia, Seaweed Max and ZR improved leaf color by 20.3%, 20.3%, and 16.9%, respectively (Figure 1). Seaweed Max generated the highest bermudagrass photosynthetic efficiency during cold stress in 2020.
Antioxidants
Antioxidant activity increased during cold stress treatment, including significantly higher activity for bermudagrass treated with Turftopia, Seaweed Max and ZR compared to the untreated control. Plants increase the production of phenolics, a broad class
of defense compounds, in response to abiotic stress. In this trial, bermudagrass exposed to chilling stress consistently responded with increased phenolic content, especially toward the end of the chilling stress exposure (data not shown). Furthermore,
on Aug. 25, 2021, and at the end of chilling stress, Turftopia and Seaweed Max treatments increased phenolic content over the untreated control by 23.5% and 18.8%, respectively.
Superoxide dismutase
Superoxide dismutase is an antioxidant enzyme that catalyzes the reaction which scavenges the superoxide ion (O2-) and converts to the harmless oxygen (O2) and hydrogen peroxide (H2O2).
In both years, SOD increased during chilling stress, including significantly higher concentration in bermudagrass leaf tissue after treatment with Turftopia and Seaweed Max (Figure 4). In 2020 and 2021 at the end of chilling stress, both the Turftopia
and MAX treated turfgrass had significantly higher SOD concentrations than the ZR and untreated controls.
Glutathione reductase
Glutathione reductase is an antioxidant enzyme that reduces glutathione, a component of Turftopia, and catalyzes the reaction that reduces dehydroascorbate, a reactive byproduct from the reduction of hydrogen peroxide. In particular, GR contributes to
a plant’s response to abiotic stress, with elevated levels an important indicator of plant oxidative stress.
GR concentration increased after bermudagrass exposure to chilling stress. In 2020, the concentration of GR increased in the untreated control 41% from Aug. 1 to 7. In 2021, when the response was more pronounced, GR increased in the untreated control
55% from Aug. 11 to 18. Seaweed Max treatment had the biggest effect on bermudagrass leaf GR concentration in both years (Table 3 – 2021 data), including significant differences from all other treatments on Aug. 18 and 25. At the end of chilling
stress, GR activity had increased 93%, 112% and 98% for Turftopia, Seaweed Max and ZR, respectively.
Glutathione
Glutathione is a powerful non-enzymatic antioxidant. GSH protects cell membranes and prevents the oxidative denaturation of proteins under stress conditions. Increased GSH concentrations would indicate heightened plant defense as a result of oxidative
stress. While the chilling stress did not cause an increase in GSH concentration, treated plants exhibited significantly higher GSH production (Figure 5).
Turftopia and Seaweed Max offered a significant boost to GSH concentrations, including a 165% and 116% respective increase in 2020 and 171% and 116% in 2021, from the start to finish of chilling stress (Aug. 11-25, 2021) (Figure 5).
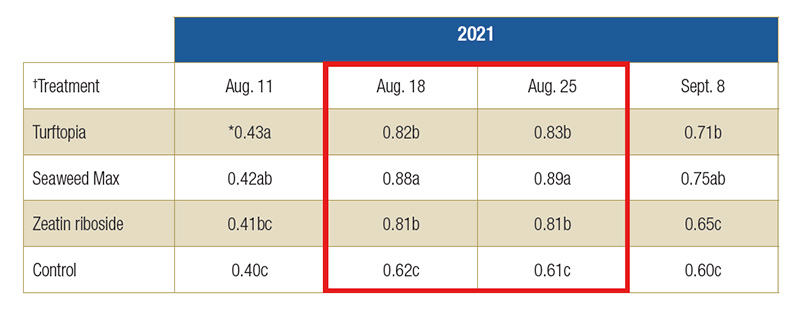
Table 3. Leaf glutathione reductase activity of hybrid bermudagrass leaf activity during and after chilling stress in enzyme units (U) per gram (fresh weight). The highlighted rows were data collected during chilling stress.
†Turftopia and Seaweed Max applied at a rate of 3.0 fluid ounces per 1,000 square feet; Zeatin riboside applied at 1.2µM
*Means followed by a different letter are significantly different (P=0.05).
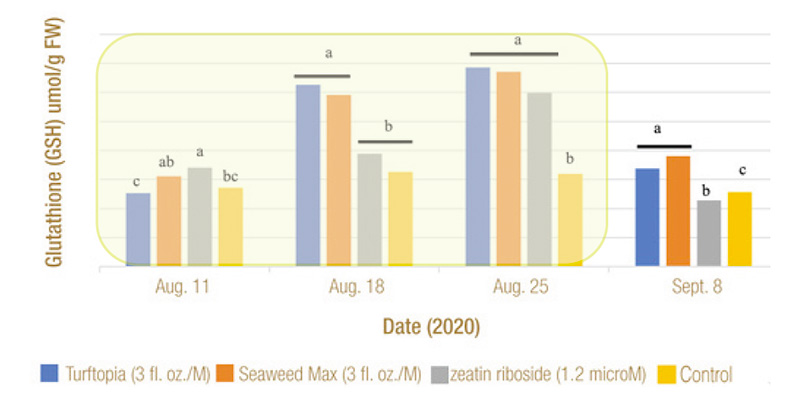
Figure 5. Effect of treatments on glutathione (GSH) concentration in bermudagrass leaf tissue on four rating dates in 2021. Chilling stress exposure occurred for 14 days (Aug. 11 to Aug. 25) as indicated by area shaded in yellow.
Means followed by a different letter are significantly different (P=0.05).
Discussion
Chilling stress slowed bermudagrass growth, reduced quality, color and photosynthetic efficiency, and increased defense pathways — including enzymatic and non-enzymatic antioxidants. Based on the results of this trial, it appears that exogenous
applications of elicitor formulations and a synthetic hormone (cytokinin) affected bermudagrass primary and secondary metabolic processes during chilling stress. It’s important to remember that four applications of each treatment were made before
the bermudagrass was exposed to chilling temperatures.
Primary metabolism
Chilling temperatures negatively affect various primary metabolic processes such as photosynthesis, respiration, energy status and water acquisition/transport. It appears that routine and preventative applications of elicitors that contain naturally occurring
cytokinin osmotic adjusters and antioxidants will relieve the chilling pressure on bermudagrass by increasing photosynthetic capacity and chlorophyll production. This was reflected in the consistent and positive color and photochemical efficiency
(Fv/Fm) response of bermudagrass to the treatments before, during and after exposure to chilling stress.
Secondary metabolism
Consistently throughout this study, treatments had a positive impact on plant defense, or secondary metabolism. Although plant defense was heightened naturally due to chilling stress, antioxidant production increased significantly for bermudagrass after
treatment with elicitors. These results corroborate previous research as it relates to increased stress tolerance of fine turfgrasses, but adds to the database of information on chilling stress of bermudagrass in a growth chamber. Given the breadth
of antioxidants affected, it appears increased defense is the primary mechanism the elicitors provided to increase bermudagrass chilling tolerance in this study. Clearly, chilling stress evokes a strong oxidative stress response, and antioxidant production
is the best plant defense.
Conclusions and recommendations
Practical implications include impacts on hybrid bermudagrasses planted and maintained into the transition zone and even the northern tier, depending upon the hybrid. Breeding has played a tremendous role to improve the cold tolerance of bermudagrass,
however additional tools including exogenous applications of plant stress reducers, or elicitors, offer a valuable tool for turfgrass managers. The treatments in this study improved the chilling tolerance of bermudagrass by extending photosynthetic
functioning and upregulating antioxidant defense. Seaweed Max, which contains naturally occurring cytokinin (Ascophyllum nodosum), had the biggest effect on bermudagrass photochemical efficiency during chilling stress. Based on these results, consider
the use of Ascophyllum nodosum as part of a comprehensive program to reduce low light and chilling stress of bermudagrass in the late fall and winter in the transition zone or northern tier. Future research might investigate these treatments in a
field setting and evaluate for winter damage.
The Research Says
- Although plant defense was heightened naturally due to chilling stress, antioxidant production increased significantly for bermudagrass after treatment with elicitors.
- The treatments in this study improved the chilling tolerance of bermudagrass by extending photosynthetic functioning and upregulating antioxidant defense.
- Seaweed Max, which contains naturally occurring cytokinin, had the biggest effect on bermudagrass photochemical efficiency during chilling stress.
- Consider the use of naturally occurring cytokinin as part of a comprehensive program to reduce low light and chilling stress of bermudagrass in the late fall and winter in the transition zone or northern tier.
Literature cited
1. Beard, J.B. 1973. Turfgrass: Science and culture. Prentice-Hall, Englewood Cliffs, N.J.
2. DiPaola, J.M., and J.B. Beard. 1992. Physiological effects of temperature stress. Pages 231-262. In D.V. Waddington et al., eds. Turfgrass. Agronomy Monograph 32. ASA, CSSA and SSSA, Madison, Wis.
3. Kauffman, G.L., D.P. Kneivel and T.L. Watschke. 2007. Effects of biostimulant on the heat tolerance associated with photosynthetic capacity, membrane thermostability, and polyphenol production of perennial ryegrass. Crop Science 47(1):261-267 (https://doi.org/10.2135/cropsci2006.03.0171).
4. Kauffman III, G.L., D.P Knievel and T.L. Watschke. 2005. Growth regulator activity of Macro-Sorb foliar in vitro. PGRSA Quarterly 33(4):134-141.
5. Levitt, J. 1980. Responses of plants to environmental stresses. Vol. 1, second edition. Academic Press, New York.
6. Liu, X., and B. Huang. 2002. Cytokinin effects on creeping bentgrass response to heat stress: II. Leaf senescence and antioxidant metabolism. Crop Science 42:466-472 (https://doi.org/10.2135/cropsci2002.4660).
7. Liu, X., B. Huang, and G. Banowetz. 2002. Cytokinin effects on creeping bentgrass responses to heat stress: I. Shoot and root growth. Crop Science 42(2):457-465 (https://doi.org/10.2135/cropsci2002.4570).
8. Sanderson, K.J., and P.E. Jameson. 1986. The cytokinins in a liquid seaweed extract: Could they be the active ingredients? Acta Horticulturae 179:113-116
9. Taiz, L., and E. Zeiger. 1998. Stress physiology. Pages 725-754. In Plant physiology, second edition, Sinauer Associations, Inc.
10. Zhang, X., K. Wang and E.H. Ervin. 2010. Optimizing dosages of seaweed extract-based cytokinins and zeatin riboside for improving creeping bentgrass heat tolerance. Crop Science 50(1):316-320 (https://doi.org/10.2135/cropsci2009.02.0090).
11. Zhang, X., and E.H. Ervin. 2008. Impact of seaweed extract-based cytokinin and zeatin riboside on creeping bentgrass heat tolerance. Crop Science 48(1):364-370 (https://doi.org/10.2135/cropsci2007.05.0262).
12. Zhang, X., and R.E. Schmidt. 1999. Antioxidant response to hormone-containing products in Kentucky bluegrass subjected to drought. Crop Science 39(2):545-551 (https://doi.org/10.2135/cropsci1999.0011183X003900020040x).
Gordon L. Kauffman III, Ph.D., (gordon.kauffman@brandt.co) is the T&O technical manager at Brandt Consolidated, Springfield, Ill., and Xunzhong Zhang (xuzhang@vt.edu), Ph.D., is associate professor-research at Virginia Polytechnic Institute and State
University, Blacksburg, Va.