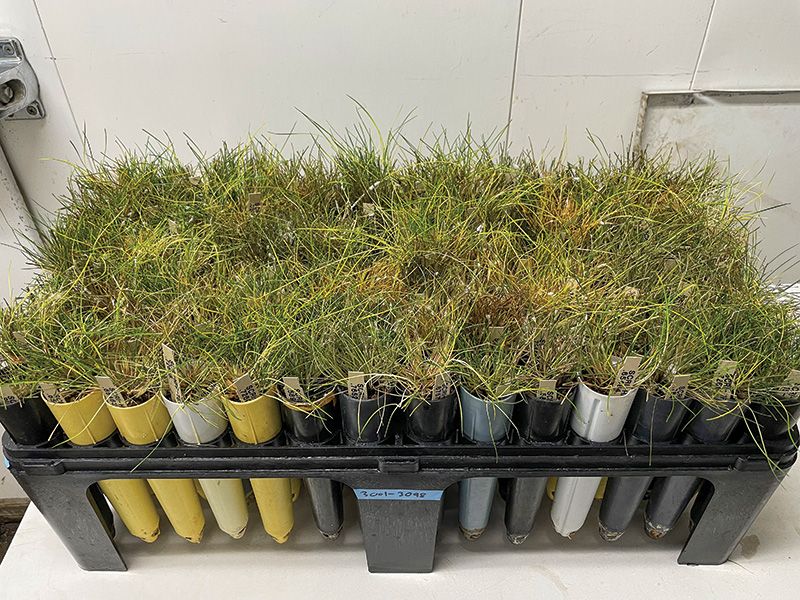
Gray snow mold affecting hard fescue plants during a growth chamber test for resistance. Photo by Paul Koch
Molecular markers have long promised to be a tool for finding valuable genes that can be selected. However, with cool-season turfgrasses like hard fescue (Festuca brevipila), finding those genes is like finding a needle in a haystack. Hard fescue has
a very large genome of about 7 billion nucleotides in size (compared to the human genome of 3 billion), is a hexaploid made up of three diploid sub-genomes and has high internal genetic variation. Genomic resources for hard fescue are sparse relative
to other turfgrass species, with some molecular markers and chloroplast sequences developed to assess species relationships (3, 5). To provide more genomic resources, leading to more robust molecular markers, we made a first-pass reference genome
assembly of the variety Beacon.
Hard fescue’s niche as a turfgrass includes areas suited for low-maintenance situations such as those with low soil fertility, areas with high heat and drought, and areas where dollar spot disease is prevalent (2). The fine fescues in general are
considered the most shade-tolerant of cool-season turfgrass species, but hard fescues are less tolerant to foliar shade in comparison to the rhizomatous red fescues (2). Gray snow mold caused by Typhula incarnata can be devastating on cool-season
turfgrasses in temperate climates around the globe, and hard fescue is not an exception (2).
Improvement for these traits would increase the utility of hard fescue as a low-maintenance turfgrass, and genome-wide association studies are a robust method to start that process. With a reference genome assembly in hand and next-generation molecular
marker technologies, we have the ability to survey the genome and find molecular markers associated with shade tolerance and snow mold resistance.
Our goal is to improve selection and improvement of these important traits in hard fescue.
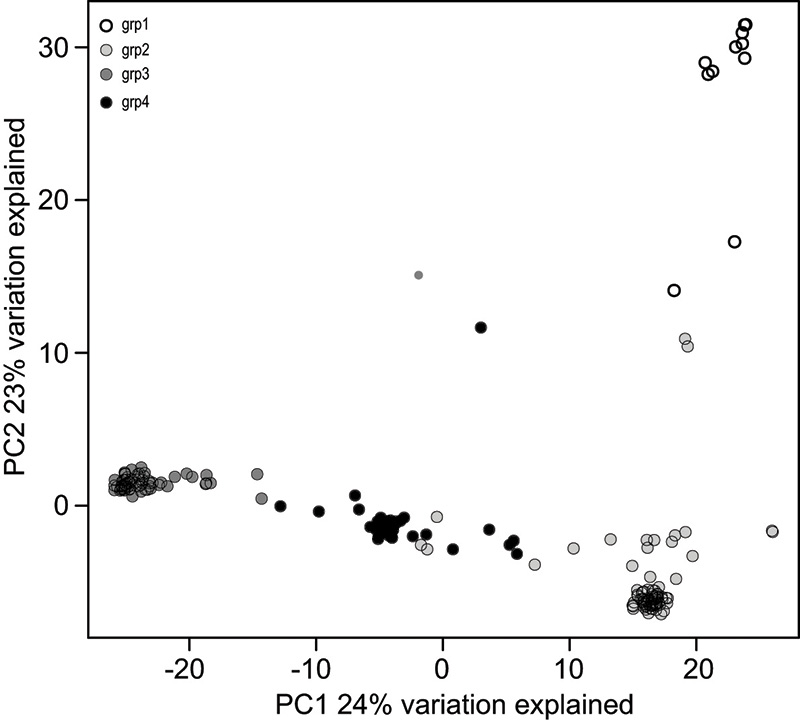
Figure 1. A principal component analysis showing a scatter of genetically structured hard fescue groups from collections throughout Eurasia.
Methods
The key to a successful genome-wide association study is the use of a diverse population; in this case, we had 240 plants derived from 75 collections housed at Rutgers University, the University of Minnesota and the National Plant Germplasm System. Seedlings
of each plant were vegetatively propagated, sampled for DNA extraction, and used for measuring growth under foliar shade and resistance to gray snow mold.
To measure the plants’ responses to simulated foliar shade, replicated clones of each plant were thinned to three tillers and moved into a simulated foliar shade tent within a greenhouse at the University of Minnesota, and photoselective filters
(one-quarter strength Cinegal 3208 color temperature blue filter and a 0.30 neutral density E-colour+ 209 filter from Rosco Laboratories Inc., Stamford, Conn.) were layered to reduce the red-to-far red (R:FR) light ratio and photosynthetic photon
flux (PPF) by approximately 70%. Light quality was measured monthly using an Apogee SS-110 spectroradiometer, while PPF was measured every five minutes with an Apogee SS-500 photosynthetic active radiation sensor. The plants were irrigated three times
each week, fertilized once per week with a complete fertilizer solution and clipped to a height of 3 inches (7.5 centimeters) every 14 days. Four traits were measured over the course of the experiment: the average 14-day regrowth throughout the four
months of treatment; the regrowth of leaves 14 days following the final clipping; the number of tillers per plant after four months of shade (at the end of the experiment); and the dry matter biomass after the final clipping at the end of the experiment.
To measure the plant responses to gray snow mold, clones of the same plants were grown to a five-tiller stage and placed in a controlled environment chamber at the University of Wisconsin-Madison. Plants were acclimated for fungal inoculations by reducing
temperature and light over 21 days from a day/night temperature of 64.4/59 F (18/15 C) and a photoperiod of 12 hours a day to a day/night temperature of 46.4/41 F (8/5 C) and a photoperiod of eight hours a day. After the acclimation period, the plants
were inoculated with Typhula incarnata collected from infected Penncross creeping bentgrass at the O.J. Noer Turfgrass Research Facility (Madison, Wis.) by placing a single infested rye grain at the base of each hard fescue plant. The racks of plants
were placed in a plastic storage container, sealed, misted with water to maintain 98% relative humidity and maintained in a controlled environment chamber at a constant temperature of 39.2 F (4 C) with no light. After six weeks, when the disease reached
the edge of the containers in the most susceptible plants, the plants were visually rated for percent disease using a categorical 0-to-6 scale, with 0 = 0% disease, 1 = 1%-10% disease, 2 = 11%-25% disease, 3 = 26%-50% disease, 4 = 51%-75% disease,
5 = 76%-90% disease and 6 = 91%-100% disease.
Because hexaploid hard fescues can and have been misclassified as diploid or tetraploid bunch-type fescues, we ensured the hexaploidy of Beacon hard fescue plants using a BD Accuri C6-plus flow cytometer (BD Biosciences, Franklin Lakes, N.J.). Genome
sequences of one of those Beacon plants were grouped and assembled into long, nearly chromosome-sized pieces, called “contigs.” Those sequences are stored in the National Center for Biotechnology Information (NCBI) under BioProject PRJNA495101
for public use. The diverse population of 240 plants was split into four partitions of 60 plants each, which were pooled and sequenced on an Illumina NextSeq 500 instrument (Illumina Inc., San Diego). The sequences were mapped to the Beacon genome
assembly to find the location of variant DNA. We found 13,230 molecular markers spread across the genome.
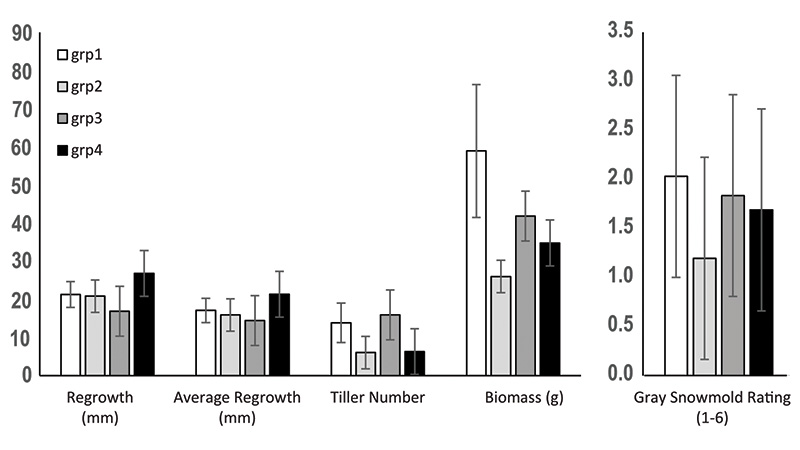
Figure 2. The shade responses and gray snow mold ratings of four genetically differentiated groups of hard fescue collections.
Results
Using a principal coordinate analysis to characterize genetic variation and relationships of the plants, the 240 plants fit into four structured groups (Figure 1). Groups 1, 2 and 4 originated primarily from Baltic regions of northeastern Europe, while
Group 3, with 69 plants, was collected from Balkan regions and Iran. The four cultivars and most of the breeding lines were included in the largest structured group of 87 plants, Group 2, from northeastern Europe. This group was characterized with
a lower biomass and tiller number under shade, yet also with a tendency for lower (better) snow mold ratings (Figure 2). Group 1 plants, on the other hand, were characterized with the highest biomass under shade but also relatively poor snow mold
ratings (Figure 2). Plant breeders can select germplasm from these groups to target their traits of interest.
For genome-wide association analysis, the molecular markers were regressed onto the shade and snow mold trait values while accounting for the population structure groups and multiple testing. Thirty-five molecular markers were significantly associated
with at least one of the four shade response traits, and three were associated with snow mold ratings (Table 1). Some of these 38 had significant but marginal effects, while others were found to be associated with more than one trait or had large
effects. One molecular marker was shared between both regrowth traits, had an effect of 1-to-1.13-inch (2.44-to-2.87-centimeter) average regrowth and was located near a cytochrome P450 encoding gene. Another marker was shared between biomass and tiller
number, was associated with an increase of 5.8 tillers under simulated foliar shade and a 0.74-ounce (21-gram) increase in biomass and was near to a molybdate transporter gene (Table 1). These markers suggest that plants grown under shade exhibit
stress responses involving cyp450 genes as well as enhanced molybdenum transport from roots to shoots. Clues from these molecular markers and their associated genes provide researchers with new possibilities for measuring and ameliorating shade stress.
Because screening for gray snow mold is challenging and trait measurement is categorical, our ability to detect variation was constrained, and only three associated molecular markers were found. However, all three had relatively large effects on snow
mold resistance, and two of them were near genes with known responses to fungal pathogens. One molecular marker was associated with a 20% difference, the second with a 16% difference, and the third was associated with 9% difference in gray snow mold
resistance. All three molecular markers for snow mold fit dominant inheritance patterns, with the reference allele from Beacon corresponding to lower resistance. Additionally, two of the three molecular markers were located near genes with plausible
roles in fungal responses: an F-box protein that is part of a large gene family involved in pathogen-triggered plant immunity (1), and a protein kinase G11A that can be involved in ABA-dependent plant stress signaling (4).
Both the shade and snow mold screenings were conducted in growth chambers, so additional associated field experiments are necessary to confirm and prioritize the molecular markers that we found. For gray snow mold in particular, field studies have the
uncertainty of years with little or no snow over a winter season. In the meantime, these molecular markers and their nearby genes are suitable to begin selection for improvement of shade tolerance and gray snow mold resistance in hard fescue.
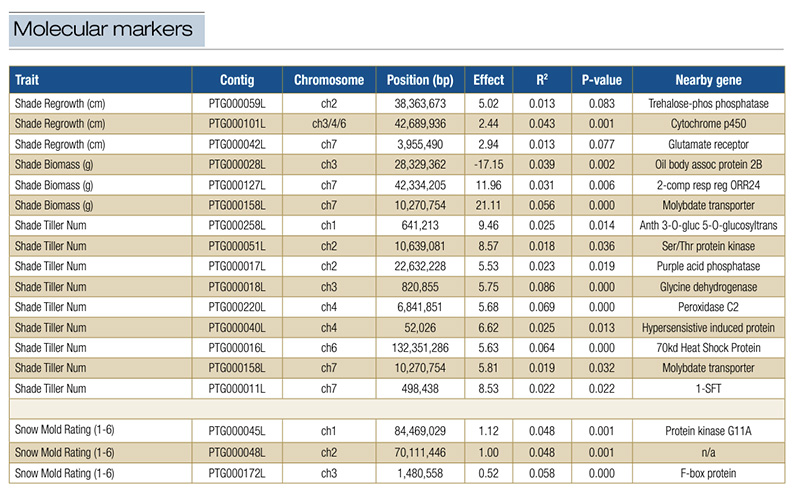
Table 1. Molecular markers associated with foliar shade response or snow mold resistance. Chromosome and contig positions are based on a first-pass genome assembly of the variety Beacon. The effect of each locus is in the units shown in column one for the respective traits.
The research says
- Both the shade and snow mold screenings were conducted in growth chambers, so additional associated field experiments are necessary to confirm and prioritize the molecular markers that we found.
- For gray snow mold in particular, field studies have the uncertainty of years with little or no snow over a winter season.
- These molecular markers and their nearby genes are suitable to begin selection for improvement of shade tolerance and gray snow mold resistance in hard fescue.
Acknowledgements
The authors wish to acknowledge the outstanding assistance of Kimberly Thorsted at the USDA-ARS Forage and Range Research Laboratory; and plant materials supplied by Stacy Bonos, Ph.D., of Rutgers University. The authors also wish to acknowledge funding
support by the National Institute of Food and Agriculture, U.S. Department of Agriculture, Specialty Crop Research Initiative under award number 2017-51181-27222.
Literature cited
- Abd-Hamid, N.-A., M.-I. Ahmad-Fauzi, Z. Zainal and I. Ismail. 2020. Diverse and dynamic roles of F-box proteins in plant biology. Planta 251:68 (https://doi.org/10.1007/s00425-020-03356-8).
- Braun R.C., A.J. Patton, E. Watkins, P.L. Koch, N.P. Anderson, S.A. Bonos and L.A. Brilman. 2020. Fine fescues: A review of the species, their improvement, production, establishment, and management. Crop Science 60:1142-1187 (https://doi.org/10.1002/csc2.20122).
- Catalán P., P. Torrecilla, J.Á.L. Rodríguez and R.G. Olmstead. 2004. Phylogeny of the festucoid grasses of subtribe Loliinae and allies (Poeae, Pooideae) inferred from ITS and trnL–F sequences. Molecular Phylogenetics and
Evolution 31:517-541 (https://doi.org/10.1016/j.ympev.2003.08.025).
- Lawton, M.A., R.T. Yamamoto, S.K. Hanks and C.J. Lamb. 1989. Molecular cloning of plant transcripts encoding protein kinase homologs. Proceedings of the National Academy of Sciences of the United States of America 86:3140-3144 (https://doi.org/10.1073/pnas.86.9.3140).
- Qiu, Y., C.D. Hirsch, Y. Yang and E. Watkins. 2019. Towards Improved Molecular Identification Tools in Fine Fescue (Festuca L., Poaceae) Turfgrasses: Nuclear Genome Size, Ploidy, and Chloroplast Genome Sequencing. Frontiers in Genetics 10:1223 (https://doi.org/10.3389/fgene.2019.01223).
Shaun Bushman (shaun.bushman@usda.gov) and Matthew Robbins are turfgrass genetics scientists with the USDA-ARS Forage and Range Research Laboratory in Logan, Utah. Yinjie Qui, Andrew Hollman and Eric Watkins are turfgrass scientists at the University of Minnesota, Department of Horticultural Science in Saint Paul, Minn. Nicole Mihelich is an applied genetics scientist at Syngenta. Dominic Petrella and Florence Breullin-Sessoms are Ohio State University research scientists in Wooster, Ohio. Ming-Yi Chou is a new scientist at Rutgers University in New Brunswick, N.J. Paul Koch is a turfgrass science lead at the University of Wisconsin in Madison.