
Figure 1. Mixed smooth crabgrass with populations controlled by and escaping control with a standard rate of quinclorac (Drive XLR8). This figure demonstrates the classic segregating population.
Digitaria spp. (crabgrasses) is consistently surveyed as one of the most common and most troublesome species of weeds to control in turfgrass (19). Most control programs emphasize the use of preemergent Group 3 herbicides like pendimethalin (Pendulum; BASF, Ludwigshafen, Germany), prodiamine (Barricade; Syngenta, Basel, Switzerland) and dithiopyr (Dimension; Corteva, Indianapolis); Group 14 herbicides like oxadiazon (Ronstar; Envu, Cary, N.C.); or Group 29 herbicides like indaziflam (Specticle; Envu, Cary, N.C.) before crabgrass emergence (8). If a crabgrass escapes a preemergence program or is identified too late for a preemergent program to be implemented, there are limited options to control emerged crabgrasses (6).
Postemergence control options for crabgrass include dithiopyr, fenoxaprop, monosodium methanearsonate (MSMA) and quinclorac. Dithiopyr has been shown to have early postemergence control, but beyond the three-leaf stage is less effective (5). Fenoxaprop, an Acetyl-CoA carboxylase inhibitor (Acclaim; Envu, Cary, N.C.), has acceptable crabgrass control up to the five-tiller stage but can injure turfgrasses in higher temperatures (3). MSMA, an organic arsenical, is known to have crabgrass control, but due to environmental concerns is now only available for limited use on golf courses and sod farms (13).
Quinclorac (3,7-dichloro-8-quinolinecarboxylic acid; Drive XLR8, BASF, Ludwigshafen, Germany) is a synthetic auxin unique for its grass-in-grass selectivity. It is currently unknown why synthetic auxin herbicides only affect broadleaf species and why quinclorac can target certain grass species as well as broadleaves (10, 16). The ability of quinclorac to selectively target specific grass species makes it a beneficial herbicide for turfgrass systems. The mode of action for quinclorac has been debated for decades: It has been positioned as a cellulose synthesis inhibitor and a synthetic auxin, and quinclorac demonstrates two different mechanisms of control between broadleaf and grass species (9, 14, 18). In broadleaf species, quinclorac acts as a typical synthetic auxin, displaying typical responses like epinasty and overgrowth when applied, while grass species exhibit a necrotic response to quinclorac applications.
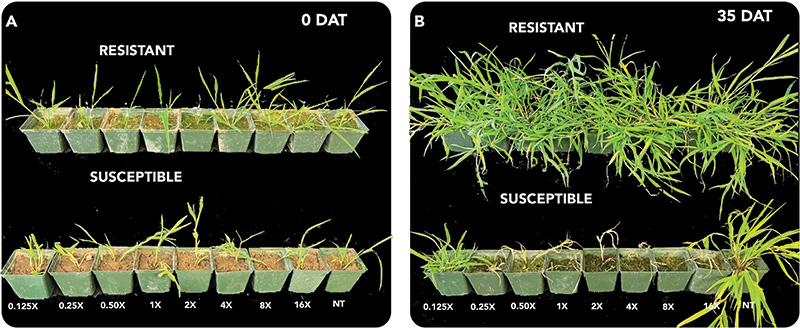
Figure 2. A) Comparison of plant injury from quinclorac application along increasing doses between AL_R1 and AL_S1 zero days after treatment. B) Comparison of plant injury from quinclorac application along increasing doses between AL_R1 and AL_S1 35 days after treatment.
Quinclorac has labeled usage in warm-season turfgrass fairways and roughs for species like bermudagrass (Cynodon dactylon), Kentucky bluegrass (Poa pratensis), buffalograss (Bouteloua dactyloides) and zoysiagrasses (Zoysia spp.). Roughs and fairways can often be contaminated by warm-season weed species like crabgrasses, barnyard grasses (Echinochloa spp.) and paspalum (Paspalum spp.), which quinclorac can control with little to no injury to the desired turfgrass species. Quinclorac is known specifically for its ability to control crabgrass, especially with small-tillered populations and mature crabgrass populations beyond the four-tiller stage. Crabgrass in the growing stages from two-tillered to four-tillered are known to escape quinclorac applications, but the reasoning behind this escape is unknown (7).
Smooth crabgrass (Digitaria ischaemum), in particular, is a warm-season annual grass weed species that has been identified as one of the most common and troublesome weeds in turf (19). Even though smooth crabgrass is an extremely common weed in turfgrass, only two types of resistance has been reported: fenoxaprop-ethyl (Group 1, inhibition of acetyl-CoA carboxylase) and quinclorac (Group 4, auxin mimics) (11).
A smooth crabgrass population (AL_R1) in Auburn, Ala., was suspected of resistance due to escaping standard field treatments of quinclorac, formulated as Drive XLR8, as seen in Figure 1. This population was discovered in a research plot with no long-term use of quinclorac, so it is unknown how the resistance trait spread to the field. A dose response assay was conducted against a known susceptible smooth crabgrass population (AL_S1) to determine the level of resistance to quinclorac.
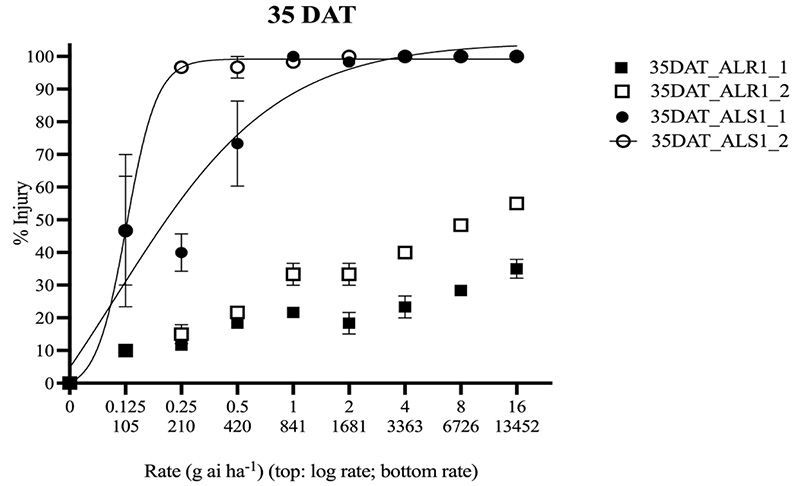
Figure 3. Percent visible injury response relative to nontreated control of smooth crabgrass populations AL_R1_1, AL_R1_2, AL_S1_1, and AL_S1_2 at 35 days after treatment. Non-log transformed rates presented for reference. Nonlinear regressions could only be modeled for AL_S1. Vertical bars represent standard error (P=0.05).
Methodology
AL_R1 was originally collected from the field in August 2022, and AL_S1 was collected in July 2023. Both populations were transplanted to the greenhouse, where each was grown to seed, and mature seed was collected, cleaned and placed in cold storage (41 F/5 C). In January 2023, AL_R1 and AL_S1 were seeded into trays with Miracle-Gro potting mix (Scotts Miracle-Gro Products Inc., Marysville, Ohio), and in March 2023 seeds began to germinate. Individual seedlings were then transplanted into 4-inch-by-4-inch (10.2-centimeter-by-10.2-centimeter) pots filled with Marvyn sandy loam. Transplanted seedlings were allowed to grow to the four-leaf stage before proceeding with the dose response assay.
A dose response screening was conducted as a randomized complete block design (RCBD). The RCBD protocol was designed using Agricultural Research Management software (ARM, GD Solutions, Brookings, S.D.) with three replicates and repeated. The initial screening for AL_R1 and AL_S1 was March 30, 2023, and the second run began April 11, 2023. Each run was kept on separate tables in the greenhouse. Foliar applications were made using a hand-held CO2-pressurized sprayer calibrated to 30 gallons per acre (280 liters per hectare) at 30 psi (206 kPa). Treatments were nine rates of quinclorac: 1.5, 3, 6, 12, 24, 48, 96 and 192 ounces active ingredient per acre (105, 210, 420, 841, 1,681, 3,363, 6,726 and 13,452 grams active ingredient per hectare) and compared to a non-treated check. These rates correspond to 0.125X to 16X the standard rate of quinclorac. The rates were log transformed into log rates to maintain equal spacing at zero for the non-treated check, and the remaining rates at 0.301, 0.602, 0.903. 1.204, 1.505, 1.806, 2.107 and 2.408, respectively. Visual injury ratings from 0% (no visible injury) to 100% (complete plant death) were collected at 7, 14, 21, 28 and 35 days after treatment (DAT). At 35 days, fresh weight was collected from each population with above-ground tissue to determine biomass reduction.
Fresh weight was transformed to a percentage based on the non-treated control for graphing purposes. Data was subjected to Analysis of Variance (ANOVA), and Fisher’s LSD ratings were calculated in R v4.3.2 using packages tidyverse v2.0.0 and agricolae v 1.3-7, while biomass reduction and dose response curves were modeled in GraphPad Prism 10 using the log (inhibitor) versus response (variable) slopes:
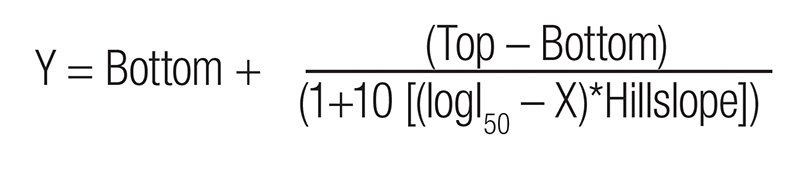
where Y is equal to injury (%), Top and Bottom are plateaus, I50 is the rate of the herbicide that gives a response halfway between the Top and the Bottom, X is the log rate of the herbicide, and Hillslope is the steepness of the curve. Model equations were selected based on best fit of commonly applied dose-response curves.
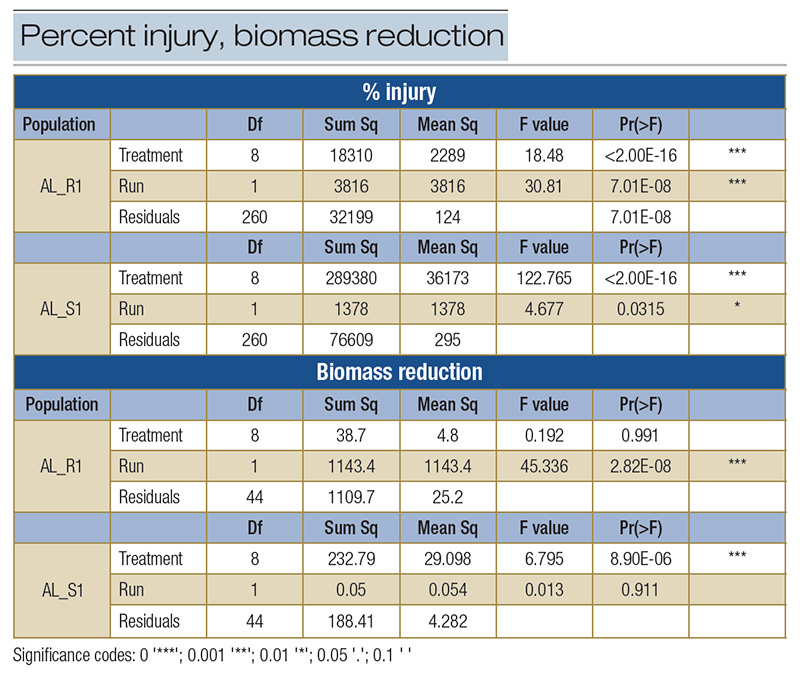
Table 1. ANOVA for dose-response study for % injury and biomass reduction. Treatment and runs for both the resistant population AL_R1 and susceptible population AL_S1 were both significantly different for % injury, thus runs could not be pooled for this analysis. For biomass reduction, run was only significantly different for AL_R1, thus AL_S1 was pooled. AL_S1 also displayed significant difference among treatments, so Fisher’s LSD values were calculated. Df: degrees of freedom; Sum Sq: sum of squares; Mean Sq: mean square; Pr(>F): p-value.
Results
The population AL_R1 was hypothesized to be resistant to quinclorac, and based on the lack of field control and visual injury, as shown in Figure 2, was confirmed resistant. At 35 DAT, all susceptible replicates were controlled 100% at the standard rate, while no resistant populations were controlled 55% at the highest rate (Figure 3). The ANOVA indicated that there was a significant difference between each run for injury response, so each run was plotted separately (Table 1). Non-linear regressions were modeled for other time points but considered redundant and thus not shown. No linear regression could be calculated for the resistant population, as 100% control was not achieved; therefore, no I50 values could be determined for the resistant population. No level of resistance could be determined as IC90 values were calculated separately, as they were not included in the model (Table 2). Fresh weights were also taken at 35 DAT. Biomass reduction was calculated relative to the non-treated population for each run. The ANOVA indicated there was a significant difference between runs for AL_R1, but not AL_S1, so biomass reduction was pooled for AL_S1. A significant interaction was present across treatment rates (P < 0.01) when calculating biomass reduction for AL_S1, but no significant treatment interaction was seen for AL_R1, as shown in Figure 4. Fisher’s LSD test groupings are also indicated in Figure 4.
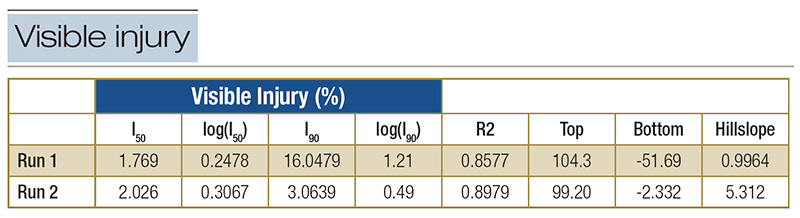
Table 2. Model parameters for susceptible population AL_S1: I50, I90 values, log equivalents, R2, Top, Bottom and Hillslope from dose-response screening for quinclorac. I50: effective concentration that gives a response halfway between Top and Bottom; log(I50): log value of I50; I90: effective concentration that gives a response at 90% between Top and Bottom; log(I90): log value of I90; R2: goodness of fit of curve; Top: the high plateau on the Y-axis; Bottom: the low plateau on the Y-axis; Hillslope: the steepness of the curve.
Discussion
The actual level of resistance to quinclorac for AL_R1 could not be calculated within the dose rate utilized. Identifying this population as resistant is interesting because the research plot where AL_R1 was found had no history of repeated quinclorac use. Resistance to quinclorac is not fully understood, but there are multiple proposed mechanisms, mostly focused on non-target-site resistance (NTSR) pathways. NTSR is a polygenic mechanism of herbicide resistance, involving the interaction of numerous metabolic systems. It has been shown that there is generally an increased accumulation of ethylene and cyanide in quinclorac-susceptible species compared to inherently tolerant ones due to the induction of ACCase (1, 9). This situation has been reported in quinclorac-resistant populations of smooth crabgrass, where there was no increase in ethylene or cyanide accumulation compared to susceptible populations (1, 17).
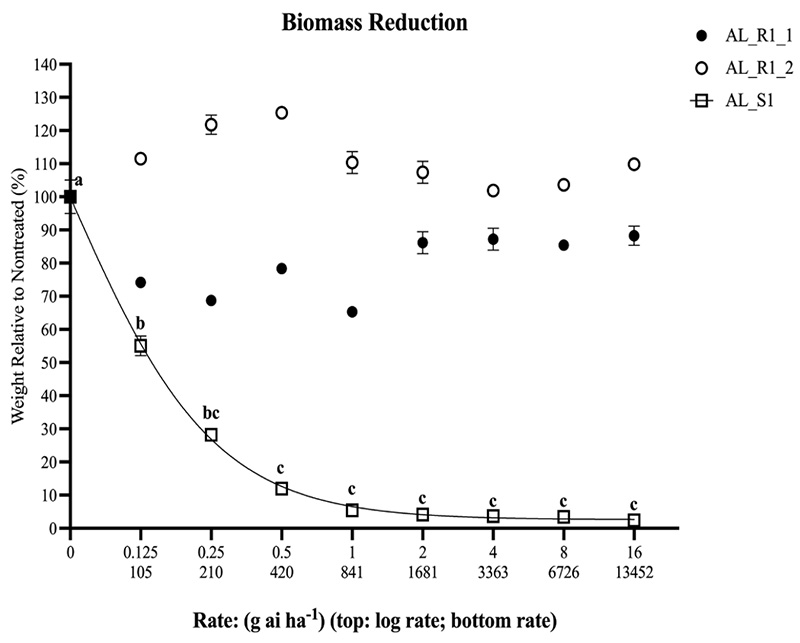
Figure 4: Biomass reduction 35 days after treatment presented as a percentage relative to the nontreated. No curve could be calculated for AL_R1_1 or AL_R1_2 as there were no differences among treatments at (α=0.05). Vertical bars represent standard error (P=0.05). Letters above AL_S1 curve indicate Fisher’s LSD groupings.
Given the high level of quinclorac resistance seen in AL_R1, it stands to reason that this resistance is likely due to a target-site mutation or another mechanism of TSR. Target-site resistance has been shown to have higher levels of resistance compared to NTSR. There are currently no mutations known to endow resistance to other synthetic auxins identified in quinclorac-resistant populations or novel mutations. However, TSR mechanisms in synthetic auxins are becoming more understood as more target-site mutations have been identified, endowing resistance to 2,4-D, fluroxypyr, dicamba and picloram (2, 15, 20).
It is currently unknown why quinclorac can selectively control smooth crabgrass, and there are some interesting taxonomic implications behind the list of species quinclorac can and cannot control. Smooth crabgrass and other similarly affected grass species are all grasses in the PACMAD clade of Poaceae, (specifically the Panicoideae subfamily), while most tolerant species are grasses in the BOP clade, as shown in Tables 3 and 4. The BOP clade is composed solely of C3 grasses, while PACMAD is composed of C3 and C4 grasses (12). The reason behind the different species presenting with susceptibility or tolerance is currently unknown. One might assume it could be associated with the differential anatomy (meaning the presence of the Kranz anatomy in C4 species) between the two subclades, as all susceptible species are C4 grasses. However, there are C4 grasses (like bermudagrass, zoysiagrass and seashore paspalum (P. vaginatum)) tolerant to quinclorac, and some C3 grasses (colonial (Agrostis capillaris) and seaside bentgrass (A. pallens)) that are susceptible.
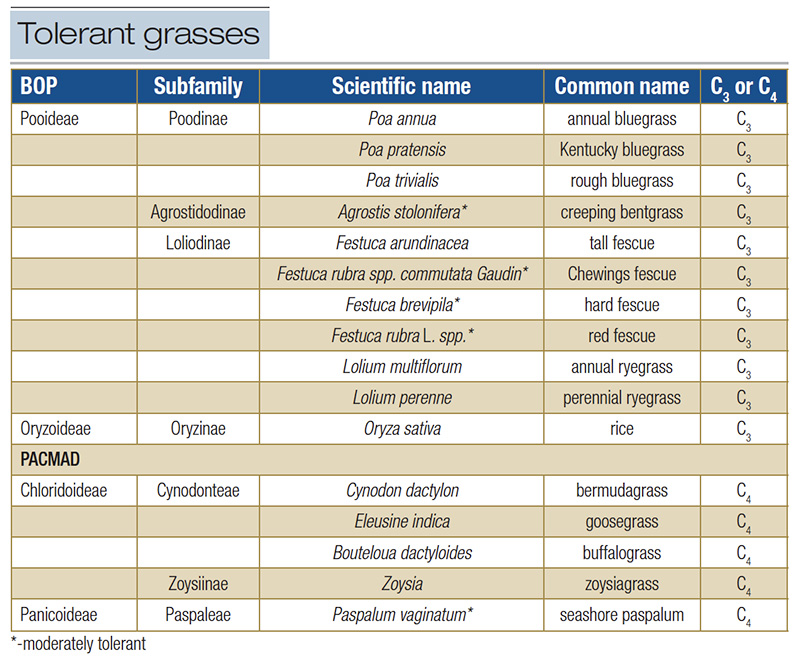
Table 3. Taxonomic classification of grasses with known tolerance to quinclorac. Species are organized by Poaceae clades, BOP or PACMAD, subfamilies, and status as a C3 or C4 species. Eleven of the 16 species are C3 grasses and five are C4 grasses.
Research Implications
More work is needed to determine if this population is resistant via a target-site or non-target-site mechanism, but it is clear that AL_R1 is resistant to quinclorac. Identification of this population further expands the growing issue of quinclorac-resistant smooth crabgrass. With quinclorac resistance becoming more prevalent, it is imperative to use resistant populations to our advantage to study how the resistance mechanism functions. Combination preemergence/postemergence programs have shown good control with other herbicides, but quinclorac is one of the few postemergence control options for crabgrass. Given the losses already incurred for crabgrass control with MSMA and the lack of effective postemergence options, ensuring the use of quinclorac for turfgrass as a control option is vital.
Finding target-site mutations for quinclorac has been particularly difficult because the target site itself is not known. However, the combination of known target-site mutations in other synthetic auxins, NTSR mechanisms for quinclorac resistance and Poaceae phylogeny can give us an idea of where we need to look next. Comparative genetic analyses between species inherently tolerant and susceptible to quinclorac may elucidate target genes responsible for increased detoxification or single nucleotide morphisms in genes previously ignored. There may be some effect that polyploidy and standing genetic variation has endowed in relation to tolerance, susceptibility or resistance to quinclorac that has yet to be uncovered.
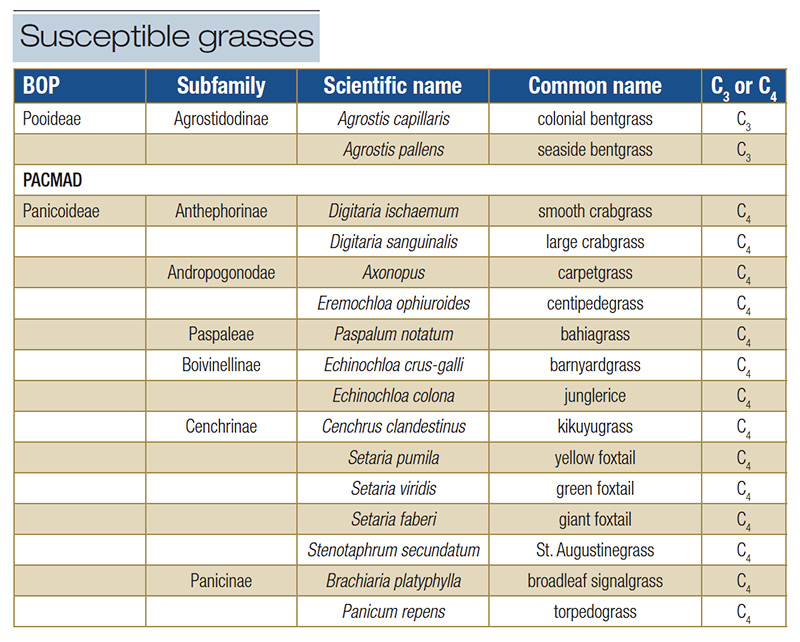
Table 4. Taxonomic classification of grasses with known susceptibility to quinclorac. Species are organized by Poaceae clades, BOP or PACMAD, subfamilies, and status as a C3 or C4 species. Two of the 16 species are C3 grasses and 14 are C4 grasses.
The research says
- More work is needed to determine if this population is resistant via a target-site or non-target-site mechanism, but it is clear that a smooth crabgrass population in Auburn, Ala., is resistant to quinclorac.
- With quinclorac resistance becoming more prevalent, it is imperative to use resistant populations to our advantage to study how the resistance mechanism functions.
- Combination preemergence/postemergence programs have shown good control with other herbicides, but quinclorac is one of the few postemergence control options for crabgrass.
- Given the losses already incurred for crabgrass control with monosodium methanearsonate (MSMA) and the lack of effective postemergence options, ensuring the use of quinclorac for turfgrass as a control option is vital.
Literature cited
- Abdallah, I., A.J. Fischer, C.L. Elmore, M.E. Saltveit and M. Zaki. 2006. Mechanism of resistance to quinclorac in smooth crabgrass (Digitaria ischaemum). Pesticide Biochemistry and Physiology 84(1):38-48 (https://doi.org/10.1016/j.pestbp.2005.05.003).
- de Figueiredo, M.R.A., A. Küpper, J.M. Malone, T. Petrovic, et al. 2022. An in-frame deletion mutation in the degron tail of auxin coreceptor IAA2 confers resistance to the herbicide 2,4-D in Sisymbrium orientale. Proceedings of the National Academy of Sciences 119(9):e2105819119 (https://doi.org/10.1073/pnas.2105819119).
- Dernoeden, P., and J. Fry. 1986. Postemergence control of crabgrass in transition zone turf using MSMA and fenoxaprop. Transportation Research Record. (https://onlinepubs.trb.org/Onlinepubs/trr/1986/1075/1075.pdf#page=4).
- Enache, A.J., and R.D. Ilnicki. 1991. BAS 514 and dithiopyr for weed control in cool-season turfgrasses. Weed Technology: A Journal of the Weed Science Society of America 5(3): 616-621 (https://doi.org/10.1017/S0890037X00027433).
- Fidanza, M.A., P.H. Dernoeden and M. Zhang. 1996. Degree-days for predicting smooth crabgrass emergence in cool-season turfgrasses. Crop Science 36(4):990-996 (https://doi.org/10.2135/cropsci1996.0011183x0036000400029x).
- Frank, K. 2022, June 29. Crabgrass control during a hot summer. Turf. (https://www.canr.msu.edu/news/crabgrass_control_during_a_hot_summer).
- Gannon, T.W., M.D. Jeffries, J.T. Brosnan, G.K. Breeden, K.A. Tucker and G.M. Henry. 2015. Preemergence herbicide efficacy for crabgrass (Digitaria spp.) control in common bermudagrass managed under different mowing heights. HortScience: A Publication of the American Society for Horticultural Science 50(4):546-550 (https://doi.org/10.21273/HORTSCI.50.4.546).
- Grossmann, K., and J. Kwiatkowski. 1995. Evidence for a causative role of cyanide, derived from ethylene biosynthesis, in the herbicidal mode of action of quinclorac in barnyard grass. Pesticide Biochemistry and Physiology 51(2):150-160 (https://doi.org/10.1006/pest.1995.1015).
- Grossmann, K., and J. Kwiatkowski. 2000. The mechanism of quinclorac selectivity in grasses. Pesticide Biochemistry and Physiology 66(2):83-91 (https://doi.org/10.1006/pest.1999.2461).
- Heap, I. 2024, May 7. The International Herbicide-Resistant Weed Database.
- Hodkinson, T.R. 2018. Evolution and taxonomy of the grasses (Poaceae): A model family for the study of species-rich groups. Annual Plant Reviews Online. Pages 255-294. Wiley, New York (https://doi.org/10.1002/9781119312994.apr0622).
- Keigwin, R.P. Jr. 2013. Organic arsenicals; amendments to terminate uses; amendment to existing stocks provisions. 46:46-2985. U.S. Environmental Protection Agency. (https://www.federalregister.gov/documents/2013/03/27/2013-07074/organic-arsenicals-amendments-to-terminate-uses-amendment-to-existing-stocks-provisions).
- Koo, S.J., J.C. Neal and J.M. DiTomaso. 1997. Mechanism of action and selectivity of quinclorac in grass roots. Pesticide Biochemistry and Physiology 57(1):44-53 (https://doi.org/10.1006/pest.1997.2258).
- LeClere, S., C. Wu, P. Westra and R.D. Sammons. 2018. Cross-resistance to dicamba, 2,4-D, and fluroxypyr in Kochia scoparia is endowed by a mutation in an AUX/IAA gene. Proceedings of the National Academy of Sciences of the United States of America 115(13):E2911-E2920 (https://doi.org/10.1073/pnas.1712372115).
- McSteen, P. 2010. Auxin and monocot development. Cold Spring Harbor Perspectives in Biology 2(3):a001479 (https://doi.org/10.1101/cshperspect.a001479).
- Putri, A.D., V. Singh, E.B. de Castro, C.A. Rutland, J.S. McElroy, T.-M. Tseng and J.D. McCurdy. 2024. Confirmation and differential metabolism associated with quinclorac resistance in smooth crabgrass (Digitaria ischaemum). Weed Science 1-9 (https://doi.org/10.1017/wsc.2024.6).
- Tresch, S., and K. Grossmann. 2003. Quinclorac does not inhibit cellulose (cell wall) biosynthesis in sensitive barnyard grass and maize roots. Pesticide Biochemistry and Physiology 75(3):73-78 (https://doi.org/10.1016/S0048-3575(03)00013-0).
- Van Wychen, L. 2023. 2023 Survey of the most common and troublesome weeds in grass crops, pasture & turf in the United States and Canada. Weed Science Society of America National Weed Survey Dataset. (https://wssa.net/wp-content/uploads/2023-Weed-Survey_Grass-crops.xlsx).
- Walsh, T.A., R. Neal, A.O. Merlo, M. Honma, G.R. Hicks, K. Wolff, W. Matsumura and J.P. Davies. 2006. Mutations in an auxin receptor homolog AFB5 and in SGT1b confer resistance to synthetic picolinate auxins and not to 2,4-dichlorophenoxyacetic acid or indole-3-acetic acid in Arabidopsis. Plant Physiology 142(2):542-552 (https://doi.org/10.1104/pp.106.085969).
Claudia Ann Landrum is a postdoctoral research associate in the Biological and Analytical Sciences Division at the Savannah River National Lab, Aiken, S.C.; Jinesh Patel is a research associate in the Department of Crop, Soil, and Environmental Sciences at Auburn University, Auburn, Ala.; James D. McCurdy is an associate professor in the Department of Plant and Soil Sciences at Mississippi State University, Starkville; Amy Wilber is an extension associate in the Department of Plant and Soil Sciences at Mississippi State University; and J. Scott McElroy is a professor in the Department of Crop, Soil, and Environmental Sciences at Auburn University.