The annual bluegrass weevil (ABW), Listronotus maculicollis, is one of the most difficult-to-control turfgrass insect pests in North America. It can cause extensive damage to short-mown turfgrass areas and has two to three generations per year, with increasing asynchrony of stages during the growing season (5). Control can be affected by widespread resistance to insecticides, often to multiple insecticide classes (1, 2, 4) and to the point where control options can be limited, particularly for adult ABW control. To reduce the selection for insecticide resistance, preventive applications against adults or small larvae should be replaced as much as possible in favor of monitoring for larvae and taking action when thresholds are exceeded.
Larval density estimates are more precise the later they are made in spring, as ABW females are capable of laying eggs over several weeks after mating (5). Most eggs will have hatched when the majority of larvae in the population are near the third instar, or approximately one week before turfgrass damage may develop. Such late decision-making requires targeting the more advanced larval stages.
Previous research has shown that cyantraniliprole, chlorantraniliprole and clothianidin are similarly effective, whether applied targeting the small larvae or third instars (1). However, it is not known how effective insecticide applications are when a significant proportion of a population has advanced to the fourth or even fifth instar. Our objective was to determine the effect of ABW larval instar, particularly of mid- to late instars, on the efficacy of insecticides available for ABW management in pyrethroid-susceptible and pyrethroid-resistant populations.
Insects
Based on previous studies (2, 3) that determined the resistance ratios of multiple populations (RR50; ratio of LD50 of tested population to LD50 of most susceptible population, i.e., population from Rutgers Horticultural Farm No. 2, North Brunswick, N.J.), susceptible (RR50 2.0: PB, Manalapan, N.J.) and resistant (RR50 55: PH, Wayne, N.J.) populations were selected for our field study.
We restricted our target application stages to the larger, more easily detectable stages, starting with the third instar. In spring, third instars start to appear in significant numbers when hybrid Catawba rhododendron (Rhododendron catawbiense) starts full bloom. At this time, ABW larval populations consist mostly of second and third instars (e.g., 1). This application target was compared with two applications made about one and two weeks later. Later application targets were not chosen because of the high likelihood that damage to the grass would start occurring.
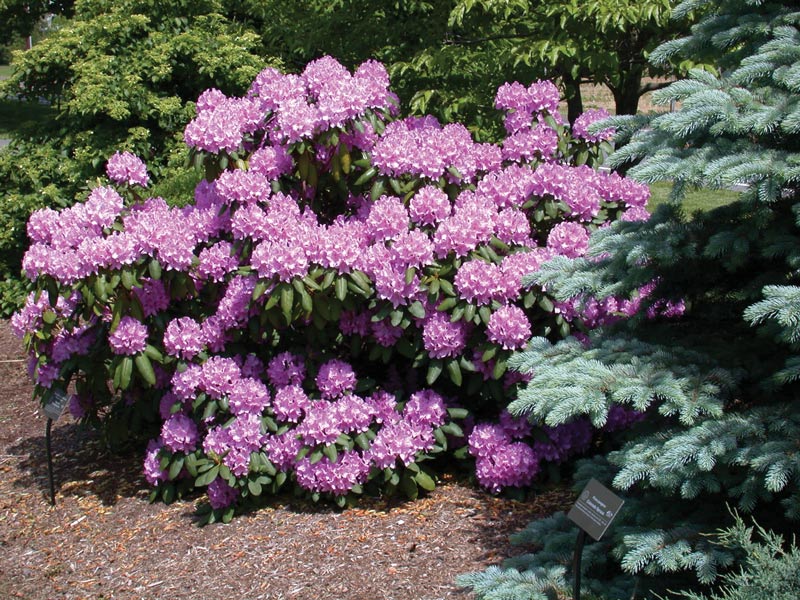
Hybrid catawba rhododendron in full bloom. Photo by R. Cowles
We labeled these application targets based on the larval instar expected at these times as determined through sampling (see below). The larval instar average (Lavg) was calculated using the following formula, where n was the number of individuals recovered of a given instar; L1 through L5 were the five larval stages; Pu was the pupal stage; TA was the teneral adult (not fully scerotized); and N the total number of all stages recovered:
Lavg = [nL1×1 + nL2×2 + nL3×3 + nL4×4 + nL5×5 + nPu×6 + nTA×7]/N
Based on the expected larval instar averages of 2.5, 3.2 and 4.0, we will in the following, for brevity, label the target application points in time as L2.5, L3.2 and L4.0 treatments or applications.
Materials and methods
Field experiments were conducted in fairway areas with a history of ABW problems at two golf courses (PB, PH) in 2017 and 2018. At PB, the grass was a mixture of 60% annual bluegrass (Poa annua), 5% creeping bentgrass (Agrostis stolonifera) and 35% Kentucky bluegrass (Poa pratensis). At PH, the grass was a mixture of 40% P. annua and 60% A. stolonifera. The grass was mowed three times per week at 0.5 inches (1.3 centimeters). Plot areas were managed by golf course staff following the same procedures as for the rest of the fairways except that no insecticides other than the experimental treatments were applied. Individual plots consisted of 3 feet × 3 feet (0.9 meters × 0.9 meters) areas separated from adjacent plots by 1 foot (0.3 meters).
A combination of growing degree day accumulation (base temperature 50 F [10 C], starting March 1; GDD50), indicator plant phenology and weekly ABW larval sampling were used to time applications. GDD50 was recorded with a WatchDog Weather Tracker Model 300. Flowering of hybrid Catawba rhododendron was observed regularly.
In each of the four experiments, there were 12 treatments consisting of one of four formulated insecticides commonly used for management of ABW larvae (4) at labeled rates (Table 1, below), each applied at one of three timings when the larval instar average was expected to be 2.5 (around 350 GDD50, onset of rhododendron full blooming), 3.2 (around 480 GDD50, rhododendron still in full bloom), and 4.0 (around 600 GDD50, late to past blooming of rhododendron), respectively.
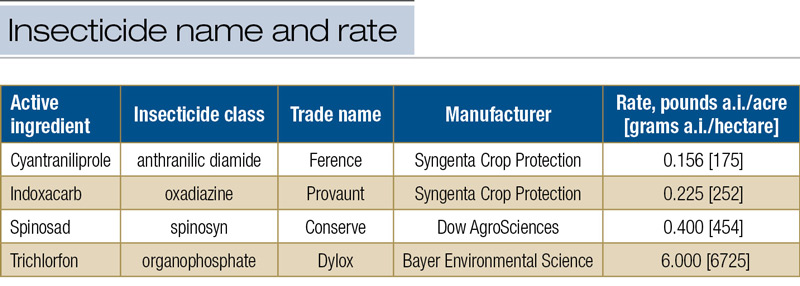
Table 1. Insecticides tested against annual bluegrass weevil larvae when larvae instar average was 2.5, 3.2 and 4.0.
Treatments were applied in 2 gallons per 1,000 square feet (823 liters per hectare) spray volume using a CO2 backpack sprayer at 30 pounds per square inch (207 kPa) pressure with a flat fan nozzle. Immediately following each application, the experiments received 0.1 inches (2.5 millimeters) of overhead irrigation to wash the insecticides onto the soil surface. Untreated control plots received only overhead irrigation. In each experiment, treatments and the untreated control were replicated six times and arranged in a randomized complete block design.
Treatments were evaluated seven days after the last application, when most individuals of the ABW spring generation were expected to be in the fourth to fifth instar and before new adults were expected to emerge from the soil. In the experiments conducted at PB in 2017 and 2018, treatments were additionally evaluated at seven days after treatment for each application timing to compare insecticide efficacy after equal time intervals.
To evaluate treatment efficacy, eight cores (2.25 inches [5.72 centimeters] diameter × 1.5-inch [3.8-centimeter] depth) were taken from each plot, and ABW stages were extracted by submerging the cores (split into four pieces) for one hour in lukewarm water saturated with table salt. Data were the combined number of larvae (all stages), pupae and teneral adults per plot. Mature adults were not included because, at this time, they were likely to originate from the overwintering generation and might have migrated into the plots from adjacent areas after residual effects of treatments had subsided.
Observations
Densities of ABW immature stages, larval stage average and stage distribution in the untreated plots at the times of application and evaluations are shown in Table 2. Population phenology followed a similar pattern across the experiments. Averaged across the four experiments, larval instar averages for the L2.5, L3.2 and L4.0 applications and the final evaluation were 2.5, 3.2, 4.0 and 4.7, respectively.
Final evaluation
Control levels were generally higher for the susceptible population (PB) but followed a similar pattern in the two populations (Figure 1, below). For the PB population, cyantraniliprole (Ference, Syngenta) applied when the larval instar average was 2.5 (cyantraniliprole-L2.5) provided the highest control, whereas cyantraniliprole-L4.0 and indoxacarb-L4.0 were the least effective treatments (Figure 2, below).
For cyantraniliprole and indoxacarb (Provaunt, Syngenta), control was significantly higher for the L2.5 and L3.2 applications than the L4.0 application. For spinosad (Conserve, Dow AgroSciences), control was significantly higher for the L3.2 than the L4.0 application, with the L2.5 application not significantly different from the other timings. Trichlorfon (Dylox, Bayer) control was significantly higher for the L3.2 than the L2.5 application, with the L4.0 application not significantly different from the other timings.
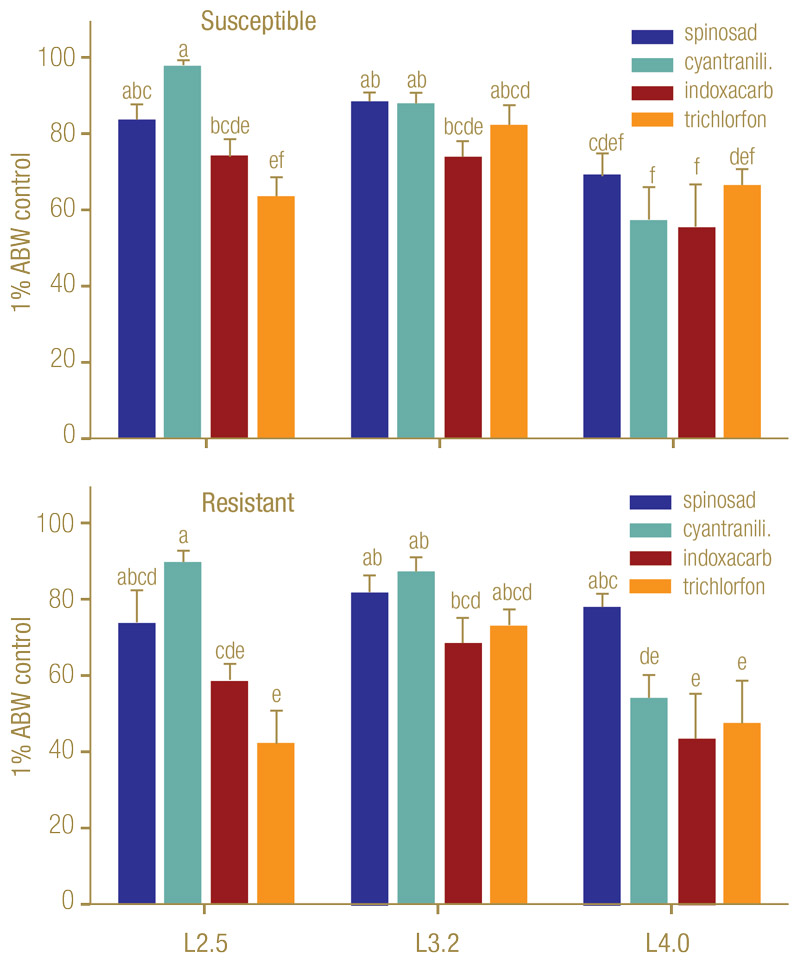
Figure 1. Effect of ABW larval instar average at the time of application on percentage control (mean ± SEM) of developmental stages with standard field rates of four insecticides in naturally infested plots on golf course fairways in spring. Experiments were conducted with a pyrethroid-susceptible (PB) (top; bifenthrin RR50 2.0) and a pyrethroid-resistant (PH) (bottom; RR50 55) population. Means with the same letter do not differ significantly among treatments within population (Tukey’s HSD, α = 0.05).
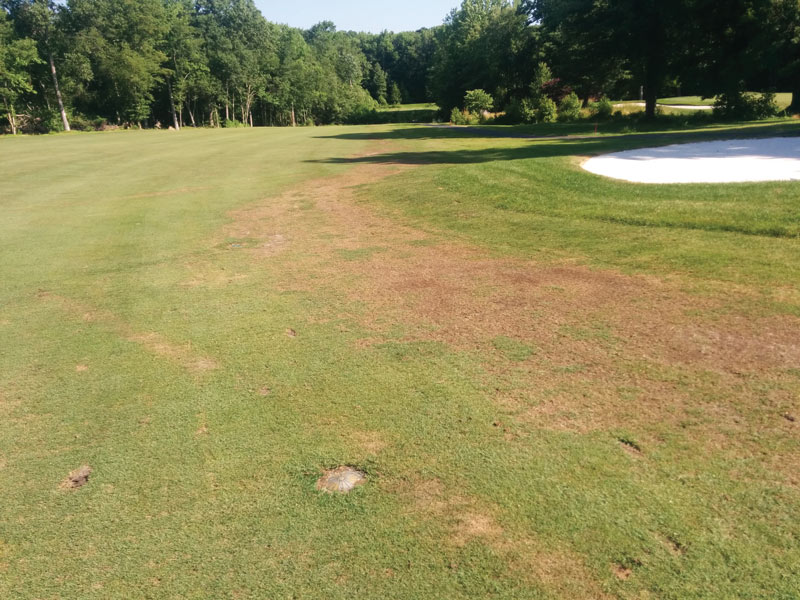
Figure 2. Late stages of damage to golf course fairways in late spring. Photo by O. Kostromytska
For the pyrethroid-resistant PH population, cyantraniliprole-L2.5 yielded the highest control, whereas trichlorfon-L4.0, indoxacarb-L4.0 and trichlorfon-L2.5 were the least effective treatments (Figure 1). For cyantraniliprole, control was significantly higher for the L2.5 and L3.2 applications than the L4.0 application. Spinosad efficacy was not significantly affected by application timing. Indoxacarb control was significantly higher for the L3.2 than the L4.0 application, with the L2.5 application not significantly different from the other timings. Trichlorfon control was significantly higher for the L3.2 than the L2.5 and L4.0 applications.
Trichlorfon was significantly less effective against the resistant than the susceptible population, whereas control by spinosad, cyantraniliprole and indoxacarb did not differ between populations.
Evaluation at seven days after treatment
For cyantraniliprole, percent control at seven days after treatment was significantly higher for the L2.5 (95%) and L3.2 applications (79%) than for the L4.0 application (57%). Trichlorfon control was significantly higher for the L3.2 application (78%) than the L4.0 application (53%), with the L2.5 application (66%) not significantly different from the other applications. Control at seven days after treatment did not differ significantly between the L2.5, L3.2 and L4.0 applications for spinosad (83%, 79% and 69%, respectively) and for indoxacarb (62%, 60% and 55%, respectively).
Overall, the increases in percent control observed at the final evaluation compared with the seven-days-after-treatment evaluation were small, indicating that for all insecticides, most larvae were killed within seven days after treatment. The increases in percent control between seven days after treatment and final evaluation for the L2.5 and L3.2 application timings, respectively, tended to be the largest for indoxacarb (14% and 11%), more variable for trichlorfon (10% and 3%) and cyantraniliprole (2% and 8%), and the smallest for spinosad (4% and 0%).
Conclusions
Our study showed that the commonly used ABW larvicides cyantraniliprole, spinosad, indoxacarb and trichlorfon provided similar levels of control of ABW larvae at the L3.2 timing as at the traditional L2.5 timing. However, the effect of application timing differed between the four insecticides tested. For cyantraniliprole and indoxacarb, the L4.0 applications were the least effective, and the L2.5 and L3.2 applications not different from each other, but this trend was stronger and more consistent in cyantraniliprole than in indoxacarb. For spinosad, there was no clear overall trend. In contrast, trichlorfon was more effective in the L3.2 than the L2.5 and L4.0 applications.
The general trend for reduced efficacy of the insecticides at the L4.0 application might be related to decreasing susceptibility as larval development advances. However, such an effect is likely modified by other factors, including changes in behavior and feeding sites of the larvae over time, differences in the distribution of the insecticides in the different parts of the grass plant, and differences in residual activity. The earlier the applications are made, the greater the proportion of young larvae that are protected from non-systemic insecticides by feeding inside the plants. In our experiments, the proportion of internally feeding larvae (first through third instars) decreased from 82% to 58% to 30% from the time of the L2.5 to the L3.2 and the L4.0 application (see Figure 1). However, the protection of these internally feeding larvae from non-systemic or weakly systemic insecticides, such as spinosad, indoxacarb and trichlorfon, may be weakened by their habit to often exit and reenter the plants, at which time they may be exposed to insecticide residues.
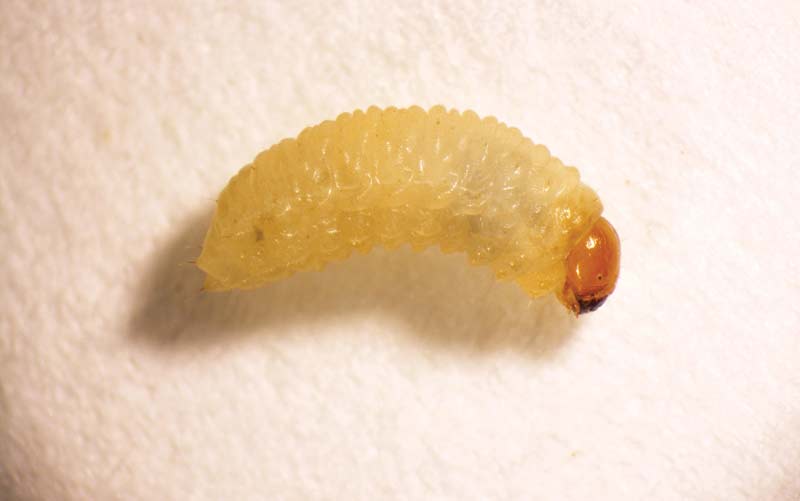
A fifth-instar larva of annual bluegrass weevil. Photo by B.A. McGraw
Our observations regarding the effect of pyrethroid resistance on insecticide efficacy were similar to previous observations (1). No effect of resistance was observed with cyantraniliprole, spinosad and indoxacarb, whereas trichlorfon was less effective against the resistant population for all timings combined.
A previous study (1) observed marginal declines in efficacy of spinosad and cyantraniliprole only against a highly resistant population (RR50 343), a decline in indoxacarb efficacy only against a highly resistant population, and a decline in trichlorfon efficacy against a resistant (RR50 95) and highly resistant population. The current study thus extended the effect of resistance on trichlorfon efficacy to an even lower resistance level (RR50 55).
Based on our observations, cyantraniliprole tends to be the most effective insecticide when applied at the commonly used L2.5 application (start full bloom rhododendron), although it was not significantly more effective than spinosad in this study. At this timing, trichlorfon was the least effective, but not significantly less effective than indoxacarb. This pattern is the same as observed previously (1) with L2.5 applications against pyrethroid-susceptible (RR50 2-2.5) and moderately resistant (RR50 30) ABW populations. At the L3.2 application timing, no significant differences were observed among the four insecticides. At the L4.0 timing, no significant differences were observed against the susceptible population, but spinosad was significantly more effective than the other three insecticides against the resistant population.
In conclusion, being able to apply the tested larvicides confidently as late as the L3.2 timing gives golf superintendents more time to monitor larval densities. Because the detection of the smallest larval stages can be challenging for the practitioner, this later timing makes it easier to accurately determine larval densities, as the proportion of small stages decreases over time. Better monitoring can then result in fewer applications being made unnecessarily. Our observations also help superintendents select the most effective insecticide if they do not recognize an infestation until large larvae are present.
Funding
This research was funded by Rutgers Center for Turfgrass Science, the Tri-State Turf Research Foundation and the USDA National Institute of Food and Agriculture Hatch Multistate project 0206130 through the New Jersey Agricultural Experiment Station (Hatch Multistate project NJ08295), Hatch project 1006804 through Pennsylvania State University, and support from Syngenta Crop Protection and Dow AgroSciences.
Acknowledgments
The authors thank the participating golf superintendents and their clubs for their cooperation. This article was based on a published paper, “Variable effect of larval stage on the efficacy of insecticides against Listronotus maculicollis (Coleoptera: Curculionidae) populations with different levels of pyrethroid resistance,” by A.M. Koppenhöfer, B.A. McGraw, O.S. Kostromytska and S. Wu in 2018 in Crop Protection (125: 2019.10488).
The research says ...
- The efficacy of the insecticides cyantraniliprole, spinosad, indoxacarb and trichlorfon for ABW control is similar whether applied when the larval instar average is L.2.5 or L3.2, but is lower at L4.0.
- At the L2.5 timing, cyantraniliprole is the most effective insecticide and trichlorfon the least effective.
- At the L3.2 timing, the four insecticides are similarly effective.
- At the L4.0 timing, spinosad is more effective than the other insecticides.
- The efficacy of trichlorfon but not of cyantraniliprole, spinosad and indoxacarb is reduced against a moderately pyrethroid-resistant ABW population.
Literature cited
- Koppenhöfer, A.M., O.S. Kostromytska and S. Wu. 2018. Pyrethroid-resistance level affects performance of larvicides and adulticides from different classes in populations of Listronotus maculicollis (Coleoptera: Curculionidae). Journal of Economic Entomology 111(4):1851-1859 (https://doi.org/10.1093/jee/toy142).
- Kostromytska O.S., S. Wu and A.M. Koppenhöfer. 2018a. Cross-resistance patterns to insecticides of several chemical classes among Listronotus maculicollis (Coleoptera: Curculionidae) populations with different levels of resistance to pyrethroids. Journal of Economic Entomology 111(1):391-398 (https://doi.org/10.1093/jee/tox345).
- Kostromytska, O.S., S. Wu and A.M. Koppenhöfer. 2018b. Diagnostic dose assays for the detection and monitoring of resistance in adults from Listronotus maculicollis (Coleoptera: Curculionidae) populations. Journal of Economic Entomology 111(5):2329-2339 (https://doi.org/10.1093/jee/toy167).
- McGraw, B.A., and A.M. Koppenhöfer. 2017. A survey of regional trends in annual bluegrass weevil (Coleoptera: Curculionidae) management on golf courses in eastern North America. Journal of Integrated Pest Management 8(1):1-11 (https://doi.org/10.1093/jipm/pmw014).
- Vittum, P.J. 2020. Turfgrass Insects of the United States and Canada, third edition. Cornell University Press, Ithaca, N.Y.
Albrecht M. Koppenhöfer is an Extension specialist in the Department of Entomology, Rutgers University, New Brunswick, N.J. Benjamin A. McGraw is an associate professor in the Department of Plant Sciences, Penn State University, University Park, Pa. Olga S. Kostromytska is an Extension assistant professor in the Stockbridge School of Agriculture, University of Massachusetts, Amherst. Shaohui Wu is a postdoctoral researcher in the Department of Entomology, University of Georgia, Tifton.